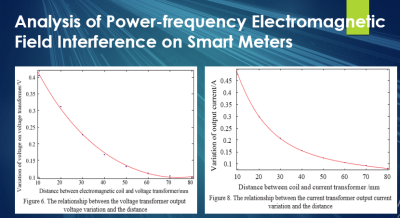
This article investigates the effects of power-frequency electromagnetic interference on key components of smart meters. Using ANSYS finite element analysis software, the study simulates and analyzes the impact of power-frequency magnetic fields (generated by a power transformer) on the smart meter’s voltage and current transformers. A finite element model is developed, and the results of the simulations are compared and analyzed. Based on these findings, the paper suggests methods for mitigating electromagnetic interference in smart meters.
Introduction:
Smart meters integrate components like power and voltage converters, current transformers, a metering chip, and a microcontroller. These devices link users and power distributors, facilitating accurate data exchange and billing through IC cards. Despite their high accuracy and durability, smart meters face growing threats from electromagnetic interference (EMI), which disrupts their operation and causes significant economic losses. EMI, often linked to power theft, generates errors in current and voltage transformers, impacting meter accuracy. Advanced tools currently struggle to detect EMI effectively on-site. In China, further efforts are needed to test and shield smart meters against EMI. Utilizing ANSYS software, this study addresses EMI issues in smart meters, analyzing their impact and proposing preventive measures.
Basic Principle
- Components of a smart meter:
- Power Supply: Provides power to the entire device.
- Voltage and Current Transformers: Convert high voltage and current to measurable levels.
- Measurement IC: (Implied) Likely involved in initial signal processing before the MCU.
- MCU (Microcontroller Unit):
- Calculates active power from voltage and current samples.
- Filters the processed data.
- Stores the results for display and transmission.
- Peripheral Circuits (LCD and Buttons): Facilitate user interaction and display information.
Working principle of transformers, voltage transformers and current transformers:
Transformers, including voltage and current transformers, operate on Faraday’s law of induction. They convert high voltage or current to smaller, measurable values or vice versa by varying the windings of their primary and secondary coils. Voltage transformers typically have more windings in the primary coil to step down voltage, with the secondary coil often connected to a voltmeter. Current transformers, conversely, reduce large currents to smaller currents, utilizing fewer primary windings. These principles underpin their critical roles in smart meter functionality and measurement accuracy.
Working principle of transformers:
A transformer includes an iron core, primary, and secondary windings, coupled magnetically but not electrically. Applying sinusoidal voltage to the primary winding generates alternating magnetic flux, inducing electromotive forces in both windings. Which is as:
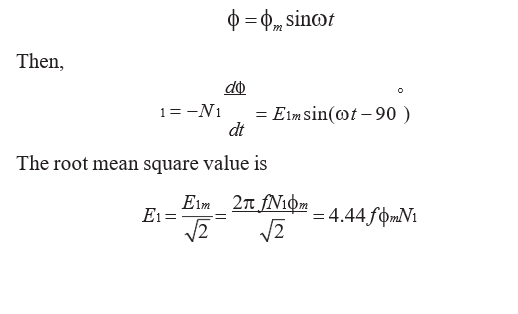
Where N1 and N2 are the numbers of the primary and the secondary windings, respectively. f is frequency of the signal. Then, the ratio of the voltage across the primary winding to the one across the secondary winding is:
The voltages in transformer windings are proportional to the number of windings. If primary voltage U1 and frequency f remain constant, the magnetic flux ϕm and induced EMF (E1) stay nearly unchanged. The total magneto motive force, combining primary and secondary contributions under load, equals the magneto motive force generated by the primary winding alone.
Because of the high permeability of magnetic core, no-load current is very small. Compared with N1I1, the current is always negligible. Therefore, we have:
The equation above shows that the currents of transformer’s primary and secondary windings are approximately equal to the reciprocal of their numbers of the turns of the windings.
Transformer modeling of Transformer without Interference:
To analyze electromagnetic interference on transformers, a 3D electromagnetic field analysis is performed using a field-circuit coupling method. Unit SOLID97 models the transformer hardware, while Unit CIRCU124 defines the circuit. An air block envelops the models, with boundary conditions ensuring zero magnetic potential at the periphery, simulating far-field effects. Proper setup of current direction in the winding coil is achieved through cylindrical coordinates. The study focuses on a single-phase transformer model, where primary and secondary coils are positioned on the same side, reducing magnetic flux leakage and improving accuracy. This approach can be extended to three-phase transformers, given their similar principles.
- Simulation on voltage transformer:
- Voltage transformers operate like regular transformers but connect the secondary side to a high-impedance measuring instrument, such as a voltmeter, effectively leaving it open-circuited.
- They step down high voltages to smaller, measurable voltages using a primary coil with more turns than the secondary coil.
- Simulation results without interference:
- Primary voltage U1=220V, secondary voltage U2=15V, with a ratio U1/U2=14.67, matching the turns ratio.
- Results with power-frequency interference:
- Adding an electromagnet changes the magnetic field strength and affects the voltage transformer’s output. The impact depends on the voltage across the electromagnet and its distance from the transformer.
- At 10mm, a 200V electromagnet causes a voltage change of 0.405V, which decreases to 0.14V at 70mm. Beyond 70mm, the impact weakens significantly.
- Increasing the coil turns or voltage across the electromagnet amplifies the interference, even at larger distances.
- Simulation on current transformer:
- Simulation Results Without Interference (Current Transformer):
- Primary current I1 = 10A, secondary current I2 = 0.683A.
- Current ratio I1/I2 = 14.64, closely matching the turns ratio N1/N2 = 14.58.
- Results with Power-Frequency Interference (Current Transformer):
- Adding an electromagnet influences the CT’s output current. The impact depends on both the electromagnet’s current (controlled by its voltage) and its distance from the CT.
- With a primary current of 10A in the CT:
- Varying the electromagnet’s voltage (50V, 100V, 200V) changes the CT’s output current.
- The relationship between the CT’s output current and the electromagnet’s current is linear.
- Increasing the distance between the electromagnet and CT (from 10mm to 80mm) reduces the electromagnet’s influence on the CT.
- The effect of the electromagnet decreases with increasing distance. At a constant electromagnet voltage, increasing the distance reduces the interference.
- Higher electromagnet current (achieved by higher voltage) results in a larger change in the CT’s output current.
General Observation:
The CT is more susceptible to interference than the voltage transformer, emphasizing the need for careful design and placement.
Power-Frequency Analysis
- Power frequency electromagnetic fields can affect the sampling of current and voltage transformers in smart meters, causing errors in electricity measurement.
- The impact depends on the strength of the electromagnetic field: stronger fields result in greater influences on both transformers.
- The influence weakens as the distance increases, but for current transformers, this change is not linear.
- The output current of current transformers is more sensitive to electromagnetic interference than that of voltage transformers.
- Shielding can significantly reduce the impact of electromagnetic fields on smart meters, using materials like iron powder to minimize external interference without increasing costs.
- Additional shielding around critical components, such as current transformers, provides further protection.
Conclusion
- Everyday electromagnetic fields (like those from power lines) can seriously mess up how accurately smart meters measure electricity, because they interfere with parts called current and voltage transformers. This can lead to wrong bills.
- Stronger electromagnetic fields cause more problems, and the closer the meter is to the source of the field, the worse the interference. Current transformers are especially sensitive.
- A good way to protect the meters is to use shielding (like a metal box) around them, especially around the current transformers. Using cheap materials like iron powder for this shielding can greatly reduce the interference.
- To make sure smart meters work reliably and give correct readings, it’s really important to design them carefully, place them in good locations, and use shielding to protect them from electromagnetic fields.