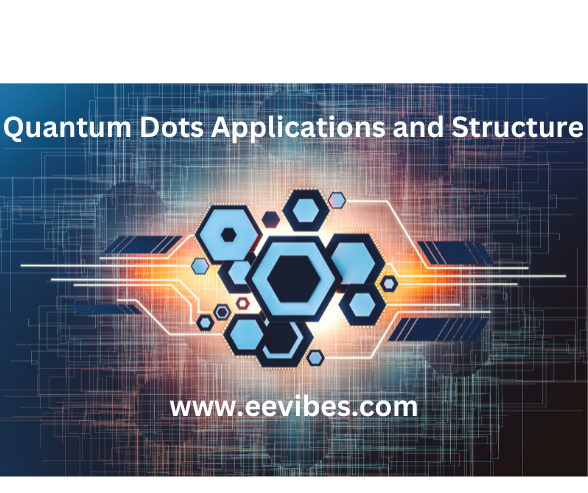
Table of Contents
Applications of Quantum Dots
What are the Applications of Quantum Dots? Quantum dots are nanoparticles that have unique optical and electronic properties due to their size-dependent quantum confinement effect. They have a wide range of potential applications in various fields, including:
- Display technology: Quantum dots can be used in display technology, such as TVs and monitors, to produce more vibrant and accurate colors.
- Solar cells: Quantum dots can be used as a light-absorbing material in solar cells to improve their efficiency.
- Medical imaging: Quantum dots can be used in medical imaging to allow for the visualization of specific tissues and cells within the body.
- Lighting: Quantum dots can be used in lighting applications to produce white light that is more energy-efficient and has a longer lifetime compared to traditional light sources.
- Sensors: Quantum dots can be used as sensors for various applications, such as detecting toxic chemicals, temperature, and pressure.
- Drug delivery: Quantum dots can be used as carriers for drug delivery, allowing for targeted and controlled release of drugs to specific areas of the body.
- Catalysis: Quantum dots can be used as catalysts in chemical reactions, which can improve the efficiency and selectivity of these reactions.
- Optoelectronics: Quantum dots can be used in optoelectronic devices, such as lasers and LEDS, to improve their performance and efficiency.
- Telecommunications: Quantum dots can be used in telecommunications to improve the efficiency and speed of data transmission.
- Data storage: Quantum dots can be used in data storage applications to increase the storage density and data transfer rate of devices such as hard drives.
Quantum Dots Fabrication
There are several methods for fabricating quantum dots, including:
- Colloidal synthesis: This is a widely used method for synthesizing quantum dots, where the nanoparticles are formed by the precipitation of semiconductor materials in a colloidal solution.
- Molecular beam epitaxy (MBE): In this method, a thin film of semiconductor material is grown layer by layer on a substrate using a beam of atoms or molecules. The film is then patterned to form quantum dots.
- Metal-organic chemical vapor deposition (MOCVD): In this method, a thin film of semiconductor material is deposited on a substrate by reacting a gas containing precursors with a metal catalyst.
- Photolithography: In this method, a patterned mask is used to selectively expose a photoresist material, which is then developed to form a patterned layer. The patterned layer is used as an etching mask to transfer the pattern to the underlying material, resulting in the formation of quantum dots.
- Self-assembled growth: In this method, quantum dots are formed through the self-assembly of semiconductor materials on a substrate.
- Nano-sphere lithography: In this method, a monolayer of polymer spheres is deposited on a substrate and then used as a template to pattern the substrate. The patterned substrate is then used as an etching mask to transfer the pattern to the underlying material, resulting in the formation of quantum dots.
Quantum Dots Structure
Quantum dots are nanoparticles that are typically composed of semiconductor materials, such as cadmium selenide (CdSe), indium arsenide (InAs), or cadmium telluride (CdTe). They have a size-dependent quantum confinement effect, which means that their electronic and optical properties are influenced by their size.
Quantum dots have a core-shell structure, with the core consisting of the semiconductor material and the shell consisting of a protective layer. The core-shell structure allows for the manipulation of the electronic and optical properties of the quantum dot by adjusting the size and composition of the core and shell.
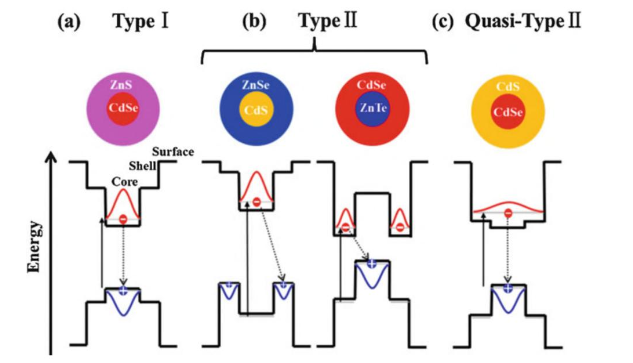
The core of the quantum dot is typically composed of a semiconductor material with a high-energy bandgap, such as CdSe or InAs. The bandgap of a semiconductor material determines the energy required to excite an electron from the valence band (the lower energy band) to the conduction band (the higher energy band). A high-energy bandgap means that more energy is required to excite an electron, resulting in the absorption of higher-energy photons.
The shell of the quantum dot is typically composed of a semiconductor material with a lower-energy bandgap, such as CdS or ZnS. The shell acts as a protective layer for the core and helps to tune the optical properties of the quantum dot.
Overall, the size and composition of the quantum dot can be precisely controlled during the synthesis process, allowing for the manipulation of their electronic and optical properties.
Also read: What are the Quantum Dots?
Quantum Dots Size
The size of a quantum dot plays a crucial role in determining its electronic and optical properties. Quantum dots are typically in the range of 2-10 nanometers in size, with the exact size depending on the material and synthesis method used.
As the size of the quantum dot decreases, the energy levels of the electrons become more closely spaced, resulting in the quantum confinement effect. This means that the electronic and optical properties of the quantum dot become size-dependent.
For example, the bandgap of a quantum dot depends on its size. As the size of the quantum dot decreases, the bandgap becomes wider, resulting in the absorption of higher-energy photons. This means that quantum dots with smaller sizes tend to absorb higher-energy photons and emit lower-energy photons, leading to a shift in the emission spectrum towards lower energies.
The size of the quantum dot also determines its quantum efficiency, which is the ratio of the number of photons emitted to the number of excitons (electron-hole pairs) generated. Quantum dots with smaller sizes tend to have higher quantum efficiencies because they have a higher density of states, which means that there are more available energy levels for the excitons to occupy.
Overall, the size of the quantum dot plays a crucial role in determining its electronic and optical properties, and it can be precisely controlled during the synthesis process.
Also read here: