Table of Contents
Introduction to Schottky diode
What is the Schottky Diode? How it is designed? As frequency is increased, the activity of small signal rectifier diodes starts to decay. They are not, at this point ready to turn off quick enough to create a well-defined half-wave signal. The answer for this issue is the Schottky diode.
Charge storage
Figure a shows a little sign diode, and Fig.b delineates its energy groups. As should be obvious, conduction-band electrons have diffused across the intersection furthermore, went into the p area prior to recombining (way A). Likewise, openings have crossed the intersection and gone into the n locale before recombination happens (way B). The more prominent the lifetime, the farther the charges can go previously recombination happens. For example, if the lifetime rises to 1 s, free electrons and openings exist for a normal of 1 s before recombination happens. This permits the free electrons to infiltrate profoundly into the p area, where they remain briefly put away at the higher energy band. Additionally, the openings infiltrate profoundly into the n area, where they are incidentally put away in the lower energy band. The more noteworthy the forward current, the bigger the quantity of charges that have crossed the intersection. The more prominent the lifetime, the more profound the entrance of these charges and the more drawn out the charges stay in the high and low energy groups. The brief stockpiling of free electrons in the upper energy band and openings in the lower energy band is alluded to as charge stockpiling. What is the Schottky Diode?
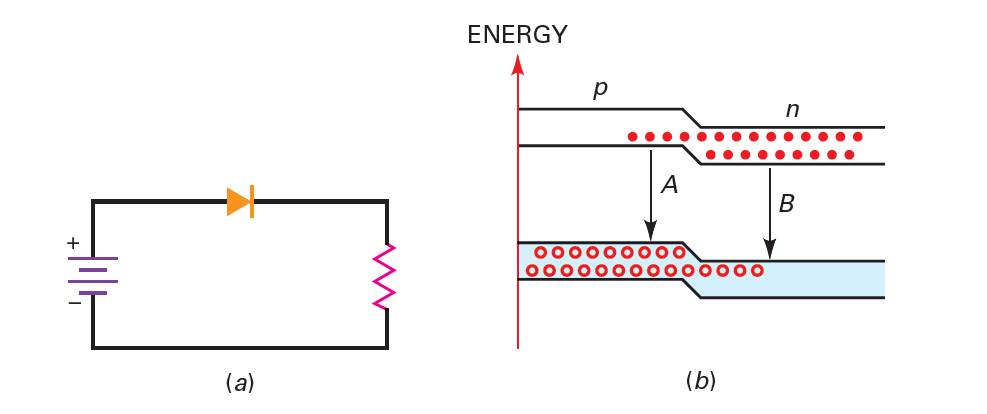
Charge Storage Produces Reverse Current
At the point when you attempt to change a diode from on to off, charge stockpiling makes an issue. Why? Since, in such a case that you out of nowhere turn around inclination a diode, the put away charges will flow the opposite way for some time. The more noteworthy the lifetime, the more drawn out these charges can add to switch current. For instance, assume a forward-one-sided diode is abruptly opposite one-sided, as demonstrated in Fig.a. At that point a huge converse current can exist for some time on the grounds that of the flow of put away charges in Fig.b. Until the put away charges either cross the intersection or recombine, the opposite current will proceed.
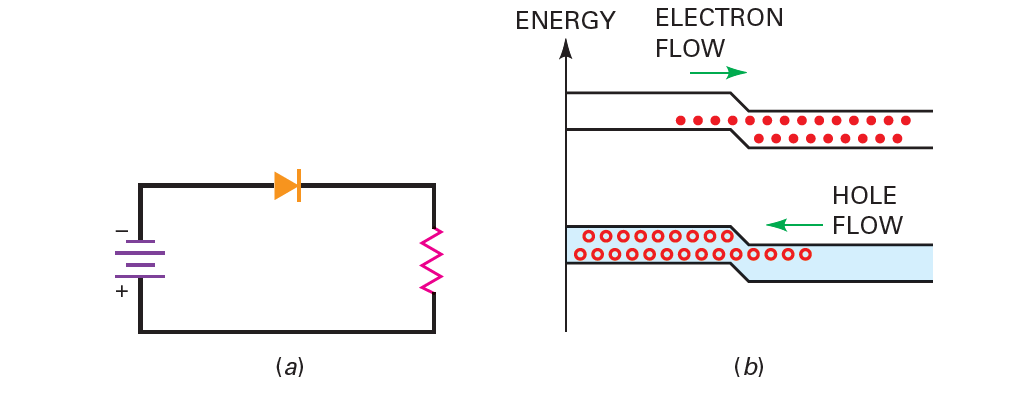
Reverse Recovery Time
The time it takes to kill a forward-one-sided diode is known as the opposite recuperation time trr. The conditions for estimating trr shift starting with one producer then onto the next. As a guide, trr is the time it takes for the opposite current to drop to 10 percent of the forward current. For example, the 1N4148 has a trr of 4 ns. On the off chance that this diode has a forward current of 10 mA and it is unexpectedly opposite one-sided, it will take around 4 ns for the opposite current to diminish to 1 mA. Turn around recuperation time is so short in little sign diodes that you don’t see its impact at frequencies beneath 10 MHz or thereabouts. It’s just when you get well over 10 MHz that you need to take trr into account.
Poor Rectification at High Frequencies
What impact does turn around recuperation time have on rectification? Investigate the half-wave rectifier appeared in Fig.a. At low frequencies, the yield is a half-wave rectified signal. As the recurrence builds well into megahertz, in any case, the yield signal starts to veer off from the half-wave shape, as demonstrated in Fig. b. Some opposite conduction (called tails) is observable close to the start of the converse half-cycle. The issue is that the converse recuperation time has become a significant some portion of the time frame, permitting conduction during the early piece of the negative half cycle. For example, if trr 5 4 ns and the time frame is 50 ns, the early piece of the opposite half-cycle will have tails like those appeared in Fig.b. As the recurrence keeps on expanding, the rectifier gets futile.
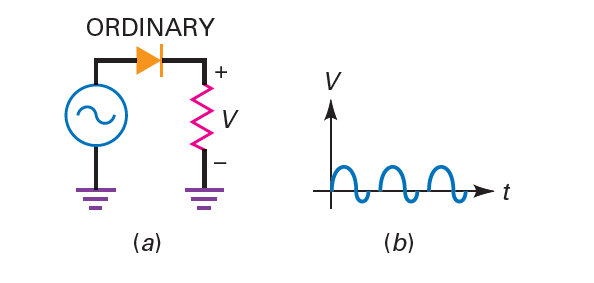
Eliminating Charge Storage
The answer for the issue of tails is a specific reason gadget called a Schottky diode. This sort of diode utilizes a metal, for example, gold, silver, or platinum on one side of the intersection and doped silicon (normally n-type) on the opposite side. Since of the metal on one side of the intersection, the Schottky diode has no exhaustion layer. The absence of an exhaustion layer implies that there are no put away charges at the intersection. At the point when a Schottky diode is unprejudiced, free electrons on the n side are in more modest circles than are the free electrons on the metal side. This distinction in circle size is known as the Schottky hindrance, around 0.25 V. At the point when the diode is forward one-sided, free electrons on the n side can acquire enough energy to go in bigger circles. Along these lines, free electrons can cross the intersection and enter the metal, creating a huge forward current. Since the metal has no openings, there is no charge stockpiling and no converse recuperation time.
Hot-Carrier Diode
The Schottky diode is now and again called a hot-carrier diode. This name came about as follows. Forward predisposition expands the energy of the electrons on the n side to a more significant level than that of the electrons on the metal side of the intersection. This increment in energy roused the name hot transporter for the n-side electrons. As soon as these high-energy electrons cross the intersection, they fall into the metal, which has a lower-energy conduction band.
Also read here:
https://eevibes.com/how-tunnel-diode-works/